Fungus Among us: implementing the bioremediation techniques of mycelium networks in water filtration
Annika Gupta Klaire Xiao
Grade 9
Presentation
Hypothesis
Objective
To investigate whether mycelium can be utilized as a natural water filtration system by testing and, comparing the pollutant removal efficiency of different mycelium species by analyzing their filtration capacity, pollutant absorption efficiency, and overall impact on water quality. This study aims to determine which species is most effective in filtering lake water, addressing the broader potential of mycoremediation for sustainable water purification.
Question
Overarching Question: Can mycelium be utilized as a natural water filteration system?
Main Problem: What species of mycelium most adeptly removes pollutants from lake water?
Hypothesis
If we filter contaminated water through filters made from three different species of mycelium, then King Oyster will be most effective because it possesses a high capacity to degrade organic pollutants and is particularly effective at absorbing heavy metals like lead and copper due to its enzymatic properties and strong adsorption capabilities, as demonstrated byt multiple previous studies.
Research
Background
1.1 What is Mycelium?
Mycelium is the mass of branched, tubular filaments, named hyphae, that make up fungi. These hyphae are typically uniform in thickness, ranging from 1 to 5 micrometers in diameter. There are two main types of hyphae: continuous hyphae, which form a single tube with multiple nuclei, and septate hyphae, which are divided by cross-walls (septa) and contain one or two nuclei per cell. This complex network of filaments allows fungi to efficiently absorb nutrients from their environment by secreting enzymes that break down complex organic materials into simpler compounds, which are then absorbed through the hyphae. These enzymes include cellulases, which break down cellulose; ligninases, which degrade lignin; and proteases, which hydrolyze proteins. This enzymatic activity is crucial for the ecological role of fungi in decomposing organic matter and recycling nutrients within ecosystems.
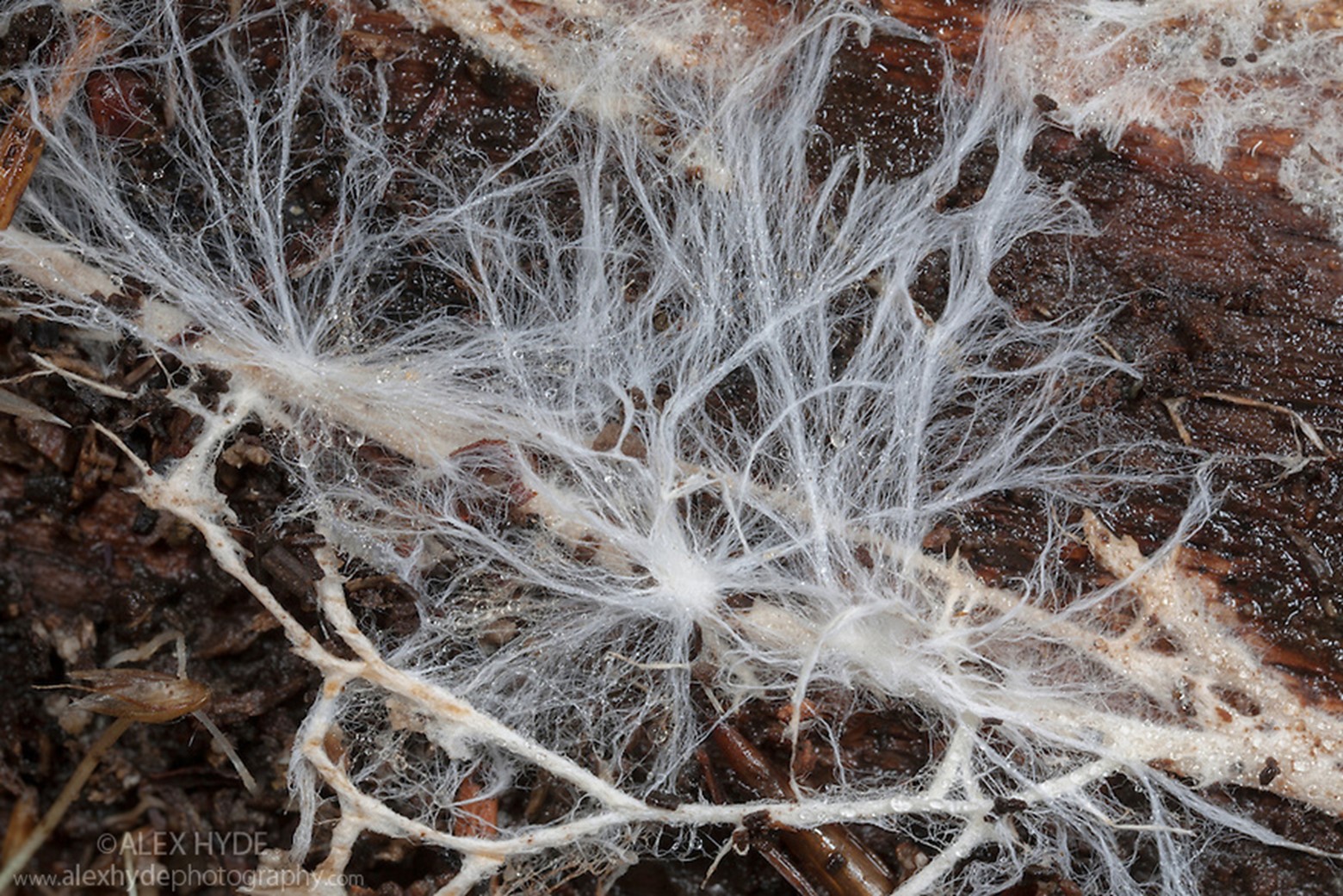
1.2 What Mechanisms Enable Mycelium to Function as a Biological Filter in Water Purification?
Mycelium purifies contaminants from water through a combination of physical filtration, absorption, and biochemical processes. The thin complex network of hyphae filaments have a high surface area that can physically trap pollutants, including dirt and other debris. In addition to this physical filtration, mycelium can absorb a variety of contaminants, particularly heavy metals like lead, cadmium, and mercury using three primary methods: biosorption, bioaccumulation, and biodegradation. In biosorption, contaminants, like heavy metals, attach to the surface of the mycelium’s hyphae through chemical interactions, allowing pollutants to stick to the mycelium without being absorbed into the cells. In bioaccumulation, the mycelium actively takes in pollutants through its cell membranes. Once inside, the contaminants can be stored or transformed into less harmful substances. Additionally, mycelium is able to break down organic pollutants, such as pesticides, through the process of biodegradation. It does so through the secretion of extracellular enzymes, such as laccases and peroxidases, which break down complex organic molecules into simpler, less toxic forms. This enzymatic action purifies the water and also transforms the pollutants into substances that are safe to be absorbed or metabolized by the mycelium. "Mycofiltration" is the name given to the application of fungal mycelium in filtering and purifying water through contaminant degradation or absorption. One of its main advantages is that it has minimal capital and maintenance expenses, as it tends to use inexpensive materials such as agricultural residue for the fungal substrate. Its systems are also based on natural biological reactions, which minimize energy-consuming mechanical processes and chemical interventions prevalent in traditional methods. However, it may be difficult to upsized mycofiltration on the large scale of application due to the likelihood of large-scale culturing and working with fungal cultures, which would remove some of the economic benefits. The lifetime of a mycofilter is also contingent on other factors, i.e., type and quantity of pollutants, existing conditions, and the applied fungus as well. Some studies show that certain mycofilters can potentially perform well for extended durations; for example, a mycofilter column did not saturate after five consecutive days of operation, showing potential for long-term application. However, other implementations, like burlap mycofiltration bags, have shown a lifespan of about one month before degradation and potential release of contaminants, indicating the importance of material selection and system design. Regular monitoring and maintenance, including possible regeneration or replacement of the fungal substrate, are essential to ensure sustained filtration efficiency. Demonstrated in multiple scenarios, this method is particularly helpful for its ability to target organic compounds, pathogens, and even heavy metals. According to a study Mycofiltration is effective at removing bacterial pathogens such as Escherichia coli and Staphylococcus aureus. The fungal cell walls and enzymes degrade harmful microorganisms, making the water safe for consumption. Additionally, mycelium can mitigate excess nutrients like nitrogen and phosphorus, which are often responsible for eutrophication in water bodies. Some fungal species can absorb heavy metals like lead, cadmium, and mercury, though this effectiveness varies by fungal strain and environmental conditions. Moreover, the enzymatic processes in fungi, particularly lignin peroxidase and manganese peroxidase, enable the breakdown of hydrocarbons, pesticides, and other persistent organic pollutants. Researchers from the University of Free State in South Africa have demonstrated that the common edible mushroom species Pleurotus ostreatus can effectively purify water contaminated with heavy metals, pathogens, and drug residues. By creating a "mycofilter" composed of the mushroom's mycelium, they achieved removal rates of up to 94% for iron (III) and 31% for the insecticide imidacloprid. This simple and sustainable method does not require special equipment or technology, making it a promising solution for improving water quality in resource-limited settings.
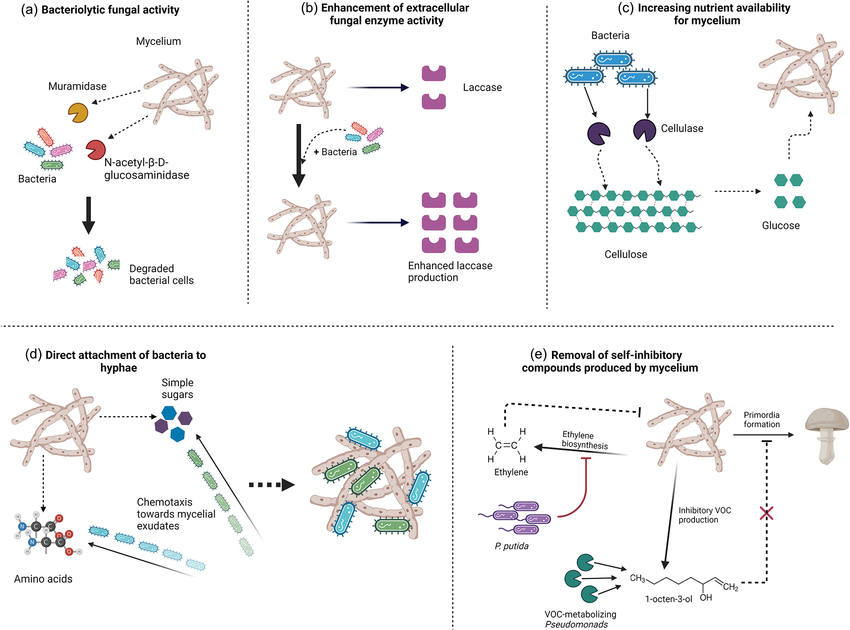
1.3 What Consititutes Aquatic Contamination and What is Its Significance?
Aquatic contamination, or water pollution, as defined by the World Health Organization, entails “water whose composition has been changed to the extent that it is unusable.” Contamination in water is a substantial issue to surrounding environments and communities, since it can lead to diseases like cholera, dysentery, and typhoid when consumed, and can contaminate vegetation through the use of unsafe water during farming. This lack of safe drinking water translates to over 500,000 deaths worldwide annually. Alarmingly, a recent study published in Science found that over 4.4 billion people in low- and middle-income countries lack access to safe household drinking water, doubling previous estimates and highlighting the severity of the crisis. Pollutants in water take many forms, including bacteria, viruses, parasites, fertilizers, pesticides, pharmaceutical products, nitrates, phosphates, plastics, fecal waste, and even radioactive substances, all of which pose severe health and environmental risks. While some contamination occurs naturally, such as mercury seeping from the Earth's crust, human activities are the main contributors to water pollution. Global warming accelerates water contamination by increasing water temperatures, reducing oxygen levels and disrupting aquatic ecosystems. Deforestation further worsens the situation by depleting freshwater sources and encouraging bacterial growth in standing water. Industrial and agricultural waste, including the dumping of chemicals, untreated sewage, and fertilizers, introduces harmful substances that make water unsafe for consumption. Shockingly, over 80% of untreated sewage worldwide is directly released into rivers and seas, contributing to the degradation of marine ecosystems and the spread of waterborne diseases. Plastic pollution from maritime activities further worsens the crisis, with microplastics contaminating water supplies and entering the food chain. With the rise in global warming and insufficient sustainability efforts, the situation is not improving but instead worsening. The 2023 United Nations World Water Development Report warns that “water pollution is worsening globally as agricultural runoff, untreated sewage, and industrial discharges increase, with rivers and lakes in Asia, Africa, and Latin America among the most impacted regions.” As pollution levels continue to rise, the need for effective policies, stronger regulations, and sustainable water management solutions becomes ever more urgent.
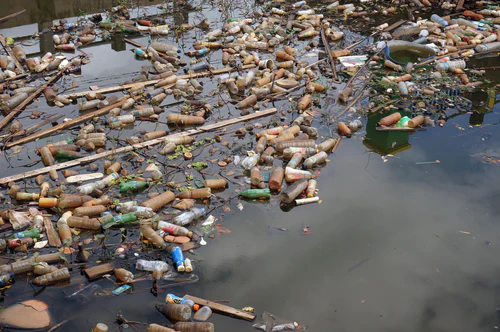
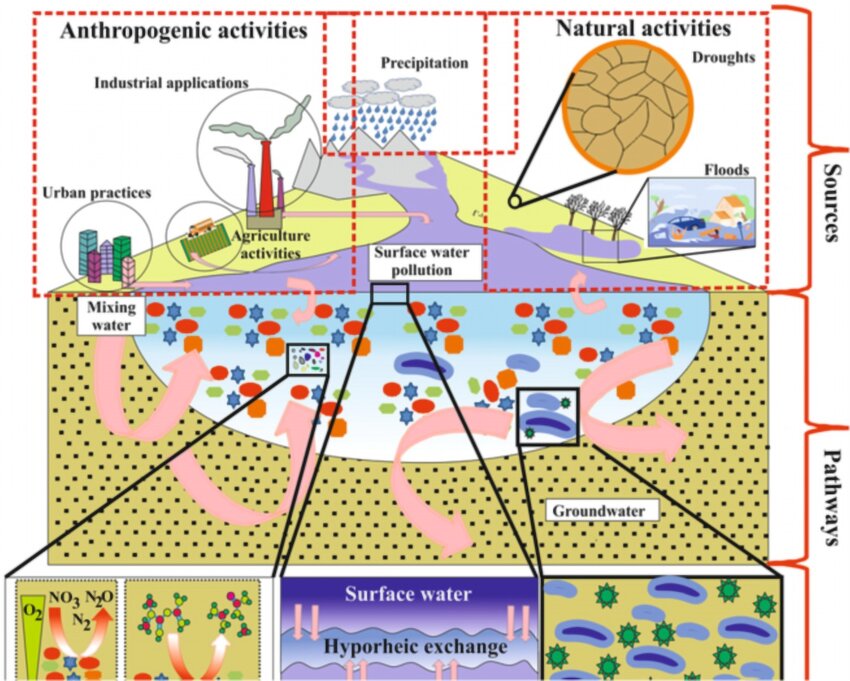
Experiment Background
2.1 What Species of Mycelium are Most Sufficent in Water Filteration?
2.3 What Substrate is Ideal in Supporting Mycelium Growth?
2.4 Filter Design
A typical water homemade water fiteration system features a plastic bottle, which holds the layers of the system together, and components like gravel, sand, and cotton, which traps larger debris and smaller suspended particles. In addition, most will include activated charcoal, a material which absorbs toxins, bacteria, and other chemicals to ensure the most sufficent removal of pollutants in the water with cost-effective and accessible materials. In our case, we avoided most other materials, since we wanted to isolate and analyze the effectivemness of the mycelium alone, and other materials, espacially such as activated charcoal could effect the result. In the previously mentioned study conducted by the University of Free State in South Africa, the team created a mycofilter by packing fungal mycelium (from Pleurotus ostreatus) into columns and contaminated water was passed through the filter to remove pollutants. Based off of this secussful pre-existing experiment, we decided to create a similar filter by packing our mycelium tightly over a cheese cloth-lined strainer and passing the water through.
Variables
Manipulated: The type of mycelium used (Pleurotus eryngii, Phanerochaete chrysosporium, Trametes versicolor).
Responding: The amount of contaminants filtered out by each type of mycelium.
Controlled:
- amount of substrates
- ratio of substrate
- date of testing
- temperature of water
- tested water
- ratio of materials temperature of mycelium environment
- duration of mycelium growth
- volume of water tested for each sample
- type and quality of equipment used for testing water parameters
- method and frequency of water parameter measurements
- preparation process for each mycelium type before testing
Procedure
Materials
- Coffee grounds (900g )
- Cardboard, cut into strips (900g)
- Mycelium cultures-Wood Rot, King Oyster, Turkey Tail (10 ML each)
- 15 plastic containers (1 L)
- Tape
- Marker
- Measuring Cup
- Oak wood chips (1200g)
- 3 plastic containers with lids (12 L)
- Cheese cloth
- Water testing strips (15 total)
- 3 metal bowls
- 20 candles
- 1 Small airtight container
- Gloves
- 70% alcohol
- Large pot
- Strainer
- Kettle
- Alcohol wipes
- Hair tie
Mycelium Cultivation
- Gather all required materials.
- Wear appropriate personal protective equipment (PPE) to ensure safety.
- Light 10 candles around the workspace to maintain a sterile environment.
- Sanitize the interior of large plastic containers using 70% isopropyl alcohol.
- Repeat the sanitization process for the container lids.
- Cut cardboard into approximately 1-inch-wide and 3-inch-long strips.
- Fill a large pot with water.
- Boil the cardboard strips at a temperature of 70–90°C for 1 hour.
- Transfer the boiled cardboard strips into a bowl and strain excess liquid.
- Drain any remaining liquid from the pot.
- Repeat steps 6–10 using oak wood chips.
- Distribute spent coffee grounds into a separate bowl.
- Boil water.
- Pour the boiling water over the spent coffee grounds.
- Loosely cover the bowl with a lid and let it sit for 1 hour.
- Strain excess water from the coffee grounds.
- Combine all prepared substrates (cardboard, wood chips, and coffee grounds) in a large bowl.
- Thoroughly mix and massage the substrate components together.
- Evenly distribute the mixture among three large containers.
- Inject mycelium cultures into the substrate.
- Repeat the inoculation process for all mycelium cultures being tested.
- Incubate the containers in a dark, warm environment for three weeks, misting with water every 3–4 days to maintain humidity.
Water Filtration and Testing
- Collect water samples from the Bow River in the Inglewood area using a large container.
- Light 10 candles around the workspace to maintain sterility.
- Remove one-third of the Turkey Tail mycelium from its container.
- Place the mycelium in a strainer lined with cheesecloth.
- Measure 1 cup of water for each filtration trial.
- Pour the water sample over the mycelium filter system, collecting the filtered water in a separate container.
- Repeat steps 25–28 for the remaining portions of Turkey Tail mycelium.
- Repeat steps 25–29 using Oyster Mushroom and Wood Rot mycelium.
- Collect control samples from tap water and unfiltered river water for comparison.
- Label all filtered and control water samples appropriately.
- Transport the samples to a certified laboratory at the University of Calgary for water quality analysis.
- Laboratory personnel conducted water quality testing using standard analytical procedures.
- Receive and review the test results provided by the laboratory.
- Record all observations and results.
- Analyze and document the data using tables and graphs.
Lighting candles to sterilize materials
Soaking substrates in water before pastuerization
Preparing water for pastuerization process
Combining the substrates
Inoculation of mycelium using liquid culture
Filteration process
Observations
Qualitative Observations:
During the mycelium growth period, we observed visible signs of colonization starting around day 4. The mycelium appeared as small, flocculent fungal spots scattered across the surface of the substrate. We also noticed that the Trametes versicolor mycelium exhibited noticeably less growth compared to the other fungi. Its rate of colonization also appeared slower, taking around 7 days to establish visible mycelium, while Pleurotus eryngii and Phanerochaete chrysosporium started showing growth after around 4 days. The Trametes versicolor mycelium appeared as thin, delicate, and slightly wispy strands spreading sparsely across the substrate. The Pleurotus eryngii and Phanerochaete chrysosporium mycelium was denser and more evenly distributed, forming thicker patches across the substrate. While growing, the boxes became humid and cloudy, with water droplets forming on the walls of the containers.
After a month, the Pleurotus eryngii exhibited plentiful mycelial growth, characterized by a dense, thick, and uniform white mat with a distinct surface layer. In contrast, the Trametes versicolor demonstrated minimal colonization, with sparse and less developed mycelial expansion compared to the other fungal species.
When collecting the water samples, we noted that the water appeared slightly cloudy, with visible sediments and dirt settled at the bottom. During the filtration process, the wood, or substrate, developed a soft, spongy texture and emitted a faint earthy smell, indicative of fungal activity. The water passed through the mycelium at a moderate rate, with an initial burst of flow that slowed over time as the substrate absorbed some water. As filtration progressed, a thin layer of sediment was visible on the surface of the mycelium, indicating the substrate's ability to trap particles. The filtered water felt slightly cooler than the input water, possibly due to the damp environment of the mycelium acting as a thermal insulator. After filtering all the water samples through the mycelium, it was noted that the colour was slightly yellow, and most sediments were not visible anymore.
Original Lake Water Sample- Floating Debris Original Lake Water Sample- Settled Debris
Water colour post-filteration Pleurotus eryngii growth after 1 month
Trametes versicolor after 1 month Phanerochaete chrysosporium after 1 month
Analysis
Sample ID |
Sample Name |
CPU/100ml Bacterial Colonies |
1 |
Original Lake Water 1 |
442 |
2 |
Orginal Lake Water 2 |
394 |
3 |
Original Lake Water 3 |
478 |
4 |
Tap Water 1 |
0 |
5 |
Tap Water 2 |
0 |
6 |
Tap Water 3 |
3 |
7 |
Wood Rot 1 |
280 |
8 |
Wood Rot 2 |
349 |
9 |
Wood Rot 3 |
308 |
10 |
King Oyster 1 |
196 |
11 |
King Oyster 2 |
187 |
12 |
King Oyster 3 |
173 |
13 |
Turkey Tail 1 |
43 |
14 |
Turkey Tail 2 |
35 |
15 |
Turkey Tail 3 |
79 |
For reference (ideal safety standards for each factor):
pH: 6.5–8.5 (EPA and WHO standards).
Carbonate, Total Alkalinity: Typically 30–150 ppm (EPA).
Lead: Below 0.01 ppm (EPA).
Nitrate: Below 10 ppm, best is 0 ppm (EPA).
Hardness: 60–120 ppm (EPA).
(Pristine Lake Water) Bacteria: 1000 CPU/ml - (GovofAB).
(Drinking Water) Bacteria: 100 CPU/ml - (GovofAB).
Analysis
Bacteria
The original lake water exhibited high bacterial contamination, with colony counts of 442 CFU/100mL in Test 1, 394 CFU/100mL in Test 2, and 478 CFU/100mL in Test 3, indicating a significant microbial presence. Tap water showed the lowest bacterial contamination, maintaining 0 CFU/100mL in Test 1 and Test 2, and only 3 CFU/100mL in Test 3, confirming its treated and sanitized nature. Among the mycelium filters, Phanerochaete chrysosporium showed moderate bacterial reduction, with colony counts of 280 CFU/100mL in Test 1, 349 CFU/100mL in Test 2, and 308 CFU/100mL in Test 3, demonstrating some variability in effectiveness. Pleurotus eryngii achieved a greater reduction, with 196 CFU/100mL in Test 1, 187 CFU/100mL in Test 2, and 173 CFU/100mL in Test 3, indicating more consistent bacterial removal. Trametes versicolor was the most effective, significantly lowering bacterial levels to 43 CFU/100mL in Test 1, 35 CFU/100mL in Test 2, and 79 CFU/100mL in Test 3, showing the highest reduction across all three tests. Overall, Trametes versicolor demonstrated the greatest bacterial removal capacity, while Phanerochaete chrysosporium was the least effective. Despite some variability, all mycelium types contributed to reducing bacterial contamination compared to the original lake water.
Carbonate
In terms of carbonate levels, the original lake water consistently measured 240 ppm across all tests. Phanerochaete chrysosporium effectively reduced carbonate levels to 180 ppm in Tests 2 and 3, while Trametes versicolor achieved a more modest reduction, lowering carbonate to 200 ppm in all tests. Pleurotus eryngii, however, did not alter carbonate levels significantly, with values remaining at 240 ppm, similar to the lake water.
pH
The original lake water had pH values ranging from 7.57 in Test 1 to 7.63 in Tests 2 and 3, indicating a slightly neutral to mildly alkaline level. Phanerochaete chrysosporium stabilized the pH at 8.03 in Tests 1 and 3 and 8.05 in Test 2, maintaining a consistent alkaline level. Trametes versicolor showed some variation, with a pH of 7.87 in Test 1, 7.76 in Test 2, and 7.77 in Test 3, while Pleurotus eryngii had a slightly wider range, with a pH of 7.85 in Tests 1 and 2, but dropping to 7.75 in Test 3. Regular tap water, by comparison, remained stable at 7.87 in Test 1 and 7.85 in Tests 2 and 3. Phanerochaete chrysosporium had the highest pH among the tested filters, while Trametes versicolor and Pleurotus eryngii both helped bring the pH closer to a neutral level. All recorded pH levels remained within the safe drinking water range established by the Government of Canada.
Lead
The lead concentration remained the same across all water samples, with results consistently showing <0.001 ppm. This suggests that the mycelium filter did not have a noticeable effect on lead levels, nor did any other factors in the experiment.
Hardness
Total Hardness
The total hardness of the original lake water samples varied across the three tests, with values of 622.7 ppm in Test 1, 658.5 ppm in Test 2, and 632.9 ppm in Test 3, indicating a relatively high mineral content. In contrast, tap water exhibited the highest hardness levels, with results of 771.3 ppm in Test 1, 794.3 ppm in Test 2, and 802.0 ppm in Test 3, confirming a significant presence of dissolved calcium and magnesium.
Among the mycelium filters, Pleurotus eryngii demonstrated the most effective reduction in hardness, with results of 620.1 ppm in Test 1, 607.3 ppm in Test 2, and 609.8 ppm in Test 3, showing a consistent decrease. Trametes versicolor resulted in slightly higher hardness levels, with values of 609.8 ppm in Test 1, 625.2 ppm in Test 2, and 602.1 ppm in Test 3, making Test 2 a slight outlier. Phanerochaete chrysosporium showed moderate reduction, with hardness levels of 681.6 ppm in Test 1, 674.7 ppm in Test 2, and 673.3 ppm in Test 3. Overall, Pleurotus eryngii was the most effective at lowering water hardness, bringing it closer, and even lowering levels to the levels observed in the original lake water. While Trametes versicolor and Phanerochaete chrysosporium also contributed to high hardness levels, tap water remained the hardest due to its high mineral content.
EDTA After Adding
The EDTA titration method was used to determine the total hardness of the water samples by measuring the amount of EDTA required to bind with the calcium and magnesium ions present in the water. The volume of EDTA added directly correlates with the hardness of the water; the more EDTA required, the higher the concentration of dissolved minerals. For the original lake water, the EDTA volume added ranged from 24.3 mL in Test 1 to 25.7 mL in Test 2 and 24.7 mL in Test 3, indicating significant but stable hardness levels. In contrast, tap water required the highest volume of EDTA, with 30.1 mL in Test 1, 31.0 mL in Test 2, and 31.3 mL in Test 3, reflecting its higher mineral concentration. For the mycelium filters, Pleurotus eryngii required the least EDTA, with values of 24.2 mL in Test 1, 23.7 mL in Test 2, and 23.8 mL in Test 3, indicating a reduction in calcium and magnesium levels. Trametes versicolor showed moderate hardness, requiring 27.7 mL in Test 1, 28.3 mL in Test 2, and 27.4 mL in Test 3, while Phanerochaete chrysosporium needed slightly higher amounts, with 26.6 mL, 27.5 mL, and 29.4 mL across the three tests. The results suggest that Pleurotus eryngii was the most effective at reducing water hardness, as it required the least EDTA to neutralize the mineral content. While Trametes versicolor and Phanerochaete chrysosporium also contributed to lowering hardness, they were slightly less effective. Tap water, requiring the highest EDTA volume, had the greatest hardness among all samples.
EDTA Before Adding
The initial EDTA volume varied slightly among the different water samples, but it remained relatively low across all tests. This is the starting point of the titration before any EDTA was added to bind with calcium and magnesium ions. For the original lake water, the initial EDTA volume ranged from 0.0 mL in Test 2 to 0.5 mL in Test 1 and 0.4 mL in Test 3, suggesting minor variations in the initial mineral content. Tap water had similar starting values, with 0.2 mL in Tests 1 and 2 and 0.5 mL in Test 3. Among the mycelium-filtered samples, Pleurotus eryngii had the lowest initial EDTA volume in Test 2 (0.0 mL) and the highest in Test 1 (0.4 mL), while Trametes versicolor remained fairly consistent at 0.2 mL, 0.1 mL, and 0.3 mL across the three tests. Phanerochaete chrysosporium showed a range from 0.0 mL in Test 1 to 0.4 mL in Test 3, indicating a slightly higher starting mineral content in some trials. These variations in initial EDTA volume indicate minor inconsistencies in mineral presence before titration began. However, since the initial values were low, they had little impact on the overall EDTA required to reach the endpoint, meaning that the total hardness results remained a reliable measure of the water's calcium and magnesium levels.
Alkalinity
Phenolphtalein Alkalinity
In all tested water samples, the number of drops of phenolphthalein alkalinity indicator remained consistently at zero across all trials, nor was any amount added in.
Bicarbonate Alkalinity
The bicarbonate alkalinity varied across all tests, with the highest values observed in tap water and the lowest in Pleurotus eryngii samples. The original water samples had moderate alkalinity, with 66 drops in Test 1, 67 drops in Test 2, and 64 drops in Test 3. Tap water exhibited the highest alkalinity, with 80, 81, and 82 drops across the three tests. In contrast, Pleurotus eryngii resulted in the lowest alkalinity, with 59 drops in Test 1, 56 in Test 2, and 54 in Test 3. Trametes versicolor showed a moderate reduction, with 70, 72, and 76 drops in each respective test. Meanwhile, Phanerochaete chrysosporium maintained relatively high alkalinity, with 79, 82, and 84 drops across the three tests. These findings indicate that Pleurotus eryngii had the most significant impact in reducing bicarbonate alkalinity, while Phanerochaete chrysosporium had the least effect, maintaining levels similar to tap water.
Total Alkalinity
The total alkalinity values varied across different water samples, with tap water and Phanerochaete chrysosporium showing the highest levels. The original water samples had total alkalinity values of 132 ppm, 134 ppm, and 128 ppm across the three tests. Tap water exhibited significantly higher alkalinity, with 160 ppm, 162 ppm, and 164 ppm. Pleurotus eryngii had the lowest total alkalinity, measuring 118 ppm, 112 ppm, and 108 ppm in the three tests. Trametes versicolor showed moderate alkalinity, with values of 140 ppm, 144 ppm, and 152 ppm. Phanerochaete chrysosporium recorded the highest alkalinity levels, with 158 ppm, 164 ppm, and 168 ppm. These results indicate that while Pleurotus eryngii effectively reduced alkalinity, Phanerochaete chrysosporium had the least impact, maintaining levels close to tap water.
Ions
Additionally, no hydroxide or carbonate ions were detected in any of the samples, indicating that the alkalinity present was primarily due to bicarbonate ions. These results suggest that tap water and Phanerochaete chrysosporium-treated water had the highest buffering capacity, while Pleurotus eryngii mycelium had the most significant reduction in bicarbonate alkalinity.
Nitrate
ppm
The nitrate concentration in the original lake water varied, with values ranging from 0.082 ppm in Test 1, 0.142 ppm in Test 2, to 0.184 ppm in Test 3, indicating some fluctuations in nitrate presence. Phanerochaete chrysosporium reduced nitrate levels to 0.076 ppm in Test 1, 0.118 ppm in Test 2, and 0.085 ppm in Test 3, showing a moderate reduction but some inconsistency across trials. Trametes versicolor also demonstrated effectiveness, lowering nitrate levels to 0.054 ppm in Test 1, 0.053 ppm in Test 2, and 0.041 ppm in Test 3. Pleurotus eryngii showed the most consistent reduction, with nitrate levels dropping to 0.059 ppm in Test 1 and 0.040 ppm in Tests 2 and 3. In contrast, tap water had the highest nitrate concentration, with values between 0.806 ppm and 0.855 ppm across tests. Both Phanerochaete chrysosporium and Trametes versicolor effectively reduced nitrate levels, making the water quality closer to ideal conditions, though not as significantly as Pleurotus eryngii, which had the most stable results.
ppm N
The nitrate-nitrogen (Nitrate-N) concentration in the original lake water fluctuated, with values ranging from 0.019 ppm N in Test 1 to 0.042 ppm N in Test 3, indicating some variability in nitrogen content. Phanerochaete chrysosporium reduced Nitrate-N levels to 0.017 ppm N in Test 1, 0.027 ppm N in Test 2, and 0.019 ppm N in Test 3, showing moderate reduction but with some inconsistency. Trametes versicolor further lowered Nitrate-N levels to 0.012 ppm N in Tests 1 and 2 and 0.009 ppm N in Test 3, demonstrating a stable and effective reduction. Pleurotus eryngii achieved the most consistent decrease, with Nitrate-N dropping to 0.013 ppm N in Test 1 and 0.009 ppm N in Tests 2 and 3. In contrast, tap water had the highest Nitrate-N concentration, maintaining values between 0.182 ppm N and 0.193 ppm N across tests. Both Phanerochaete chrysosporium and Trametes versicolor significantly reduced nitrogen content, making the water quality closer to ideal conditions, though Pleurotus eryngii showed the most stable and effective reduction.
Implication of Results
Includes:
- Interpretation of numerical values (explain what the numbers mean)
- Implications (what the results suggest or how they can be applied)
- Reasoning (why these results occurred, scientific explanation)
- Supported ideas (how these findings align with or support existing knowledge, theories, or applications)
Bacteria
The bacterial colony counts from the tests demonstrate that Trametes versicolor is the most effective in reducing bacterial contamination in water. This outcome is primarily attributed to the antimicrobial properties of Trametes versicolor, which contains bioactive compounds such as polysaccharides, phenolic compounds, and triterpenoids. These compounds have been shown in numerous studies to possess bactericidal and bacteriostatic effects, inhibiting the growth of various pathogenic bacteria, including E. coli and Staphylococcus aureus. Furthermore, Trametes versicolor produces enzymes like laccases and peroxidases, which are capable of breaking down bacterial cell walls and biofilms, crucial for eliminating bacterial contamination. The relatively high reduction in bacterial colonies observed with Trametes versicolor can be linked to these compounds’ ability to interact with and disrupt microbial cells. In comparison, Pleurotus eryngii, although effective in breaking down organic pollutants, lacks the same antimicrobial potency. It produces bioactive compounds, but they are more geared towards degrading organic material, rather than specifically targeting bacterial pathogens. Similarly, Phanerochaete chrysosporium showed moderate reductions in bacterial colonies, but its enzymatic activity is more specialized in breaking down lignin and cellulose, not bacterial cells. These findings are consistent with previous studies that support the effectiveness of Trametes versicolor in water filtration for bacterial removal, while highlighting Pleurotus eryngii’s role in pollutant degradation and Phanerochaete chrysosporium’s involvement in the breakdown of organic materials. Thus, Trametes versicolor emerges as the most effective option for bacterial filtration in water, whereas Pleurotus eryngii is more suited to addressing mineral content and hardness.
Carbonate
Phanerochaete chrysosporium demonstrated the strongest reduction in carbonate concentrations due to its ligninolytic enzymes, which break down complex compounds and help regulate alkalinity, preventing mineral buildup and scaling in water systems. Trametes versicolor also contributed to lowering carbonate levels, though to a lesser degree, indicating differences in enzymatic activity and interaction with dissolved minerals. In contrast, Pleurotus eryngii had no significant impact on carbonate concentrations, as its enzymatic processes primarily target organic matter rather than mineral filtration.
pH
The original lake water exhibited a neutral to mildly alkaline pH, while Phanerochaete chrysosporium consistently increased and stabilized pH at a more alkaline level. In contrast, Trametes versicolor and Pleurotus eryngii showed minor fluctuations, bringing the pH closer to neutral but with less stability. Tap water remained steady throughout testing, reflecting its controlled treatment for human consumption. Phanerochaete chrysosporium demonstrated the strongest buffering effect, likely due to its biochemical activity in either releasing alkaline compounds or neutralizing acidic ones. Meanwhile, the variability in Trametes versicolor and Pleurotus eryngii suggests they interact differently with water chemistry. Despite these differences, all tested water samples remained within safe drinking water standards, reinforcing the idea that mycelium-based filtration can influence water quality and potentially serve as a natural treatment method.
Lead
The lead concentration remained unchanged across all tested water samples, indicating that the mycelium filter did not have a measurable impact on reducing lead levels. This consistency suggests that the initial lead concentration was already minimal, leaving little to be removed. Another possibility is that mycelium may not be particularly effective in binding or filtering lead under the conditions used in this experiment. Since lead contamination in water can pose significant health risks, further testing with higher lead concentrations or alternative mycelium species could help determine whether it has any potential as a natural filtration method. Additional research could also explore modifications to the filtration process to enhance lead removal efficiency.
Hardness
Total Hardness
The total hardness of the water samples varied, with tap water exhibiting the highest mineral content, followed by the original lake water. Among the mycelium filters, Pleurotus eryngii demonstrated the most effective reduction in hardness, consistently lowering levels closer to those observed in the lake water. Trametes versicolor showed moderate effectiveness, with a slight outlier in Test 2, while Phanerochaete chrysosporium exhibited the least reduction. This suggests that Pleurotus eryngii may be the most efficient natural filter for softening water. The varying effectiveness can be attributed to each mycelium species' ability to adsorb and remove calcium and magnesium ions, which contribute to water hardness. These results support the potential of mycelium filtration as a sustainable method for water treatment, though its efficiency depends on the species used. Pleurotus eryngii may possess a higher affinity for binding these minerals, leading to greater reductions in hardness, whereas Trametes versicolor and Phanerochaete chrysosporium may have lower ion-binding capabilities.
EDTA After Adding
The EDTA titration method was used to assess the total hardness of the water samples by measuring the volume of EDTA required to bind with the calcium and magnesium ions present. Higher EDTA volumes correspond to greater hardness, as more EDTA is needed to neutralize the dissolved minerals. The original lake water showed stable but significant hardness, with a consistent volume of EDTA added across the tests. Tap water had the highest hardness, requiring the most EDTA, reflecting its higher mineral concentration. Among the mycelium filters, Pleurotus eryngii was the most effective at reducing hardness, requiring the least EDTA to neutralize the minerals. Trametes versicolor and Phanerochaete chrysosporium showed moderate effectiveness in lowering hardness, but to a lesser extent. These results suggest that Pleurotus eryngii is the most efficient at reducing water hardness, likely due to its ability to absorb and remove calcium and magnesium ions. The variation in effectiveness among the mycelium species highlights the potential for using specific mycelium types for water softening, while tap water’s high mineral content makes it harder to treat.
EDTA Before Adding
The initial EDTA volumes across the water samples showed slight variation, but they remained relatively low across all tests. These initial values represent the starting point before EDTA was added to bind with calcium and magnesium ions, indicating the baseline mineral content of the water. The inconsistencies observed in the starting values suggest small variations in the mineral composition of each sample before titration began. However, these variations did not significantly influence the total amount of EDTA required to reach the endpoint, meaning they had little impact on the overall hardness measurement. The slight fluctuations in the starting EDTA volume can be attributed to natural differences in mineral content across the samples, but they did not affect the comparison between the mycelium-filtered samples and the tap water. These findings support the idea that small variations in initial mineral content are expected in water analysis, but they do not significantly alter the reliability of hardness testing using EDTA titration. The results align with existing methods of measuring water hardness and demonstrate the usefulness of EDTA titration in quantifying calcium and magnesium levels in different water sources.
Alkalinity
Phenolphtalein Alkalinity
The lack of phenolphthalein indicator usage across all tested water samples suggests that the water samples had low or no alkalinity that could cause a noticeable color change in the presence of phenolphthalein. Phenolphthalein is typically used to detect the presence of basic substances, turning pink in alkaline conditions. Since no drops of phenolphthalein were added in any of the trials, it indicates that the water samples did not have a significant concentration of alkaline substances that would require neutralization by a titrant. This result implies that the water samples tested were not highly alkaline, aligning with expectations for natural water sources and filtered samples. The lack of change further suggests that the mycelium filters did not significantly alter the alkalinity of the water. This could be because the mycelium itself did not release enough basic substances into the water to affect its alkalinity levels. The findings support the notion that phenolphthalein is a reliable indicator for detecting alkaline substances and that none of the water samples had a high enough concentration of alkaline ions to require the use of phenolphthalein in the titration process.
Bicarbonate Alkalinity
The results for bicarbonate alkalinity demonstrate clear variation across the water samples, with tap water exhibiting the highest alkalinity and Pleurotus eryngii the lowest. Alkalinity, primarily driven by bicarbonate concentrations, plays a crucial role in maintaining pH stability in water. The higher alkalinity in tap water suggests a significant presence of bicarbonates, which serve as a buffer, preventing large shifts in pH. In contrast, Pleurotus eryngii demonstrated a capacity to lower bicarbonate alkalinity, possibly through biochemical processes that interact with and reduce the levels of dissolved compounds. The original lake water showed moderate alkalinity levels, reflecting the natural variation typically observed in environmental water sources, influenced by local conditions such as soil and runoff. Trametes versicolor and Phanerochaete chrysosporium both exhibited intermediate effects on alkalinity, with Trametes versicolor slightly reducing the levels and Phanerochaete chrysosporium maintaining higher alkalinity close to that of tap water. This indicates that different mycelium types may impact water chemistry in distinct ways, potentially due to their varied metabolic processes. These findings suggest that mycelium has a measurable effect on water’s buffering capacity, which may have implications for water treatment applications, particularly in controlling pH fluctuations in different water systems.
Total Alkalinity
The total alkalinity values of the water samples reveal significant variation, with tap water and Phanerochaete chrysosporium displaying the highest levels. Alkalinity is a key indicator of water’s ability to neutralize acids and maintain pH balance, primarily determined by the concentration of bicarbonates, carbonates, and hydroxides in the water. Tap water consistently exhibited the highest alkalinity, likely due to dissolved minerals and additives that enhance its buffering capacity.
In comparison, the original water samples showed moderate alkalinity, reflecting typical natural concentrations of these minerals. The Pleurotus eryngii samples demonstrated a consistent reduction in total alkalinity, suggesting that this particular mycelium type may have properties capable of lowering the buffering capacity of water. This could be attributed to its potential interactions with the dissolved minerals, possibly through processes like absorption or chemical reactions that reduce alkalinity. Conversely, Phanerochaete chrysosporium had the least effect on alkalinity, maintaining levels comparable to tap water across all tests. This suggests that Wood Rot Mycelium may not significantly influence water chemistry in the same way as Pleurotus eryngii. The moderate alkalinity observed in Trametes versicolor indicates that it has a lesser but still noticeable impact on alkalinity, falling between the effects of Pleurotus eryngii and Phanerochaete chrysosporium. These results highlight the varying influence of different mycelium types on water's alkalinity, providing insights into their potential applications in water treatment processes.
Ions
The absence of detectable hydroxide or carbonate ions in all water samples indicates that the observed alkalinity was primarily attributed to bicarbonate ions. Bicarbonates are the dominant contributors to alkalinity in natural waters, serving as the primary buffering agents to maintain pH stability. This suggests that in the tested samples, bicarbonate ions played a central role in buffering the water, particularly in tap water and Phanerochaete chrysosporium-treated samples, which exhibited the highest buffering capacity. The elevated alkalinity in these samples shows an ability to resist pH fluctuations, making them more stable in terms of acid-base balance. In contrast, the Pleurotus eryngii-treated water demonstrated the most significant reduction in bicarbonate alkalinity, indicating that this mycelium type may have had an effect on bicarbonate ions, potentially through absorption or chemical interactions that reduced their concentration. This suggests that Pleurotus eryngii may actively influence water chemistry by interacting with bicarbonate ions, potentially lowering the water's ability to buffer pH changes. These findings provide further insight into the mechanisms by which mycelium can alter water chemistry, specifically in terms of its impact on alkalinity and buffering capacity. This could have implications for water treatment processes that require adjustments to pH stability.
Nitrate
ppm
The nitrate concentration in the original lake water showed variability, with values fluctuating slightly across the tests. Phanerochaete chrysosporium contributed to a moderate reduction in nitrate levels, although the reduction was not consistent across all trials. Trametes versicolor also proved effective in lowering nitrate levels, with a notable reduction across all tests. Pleurotus eryngii demonstrated the most consistent and significant decrease in nitrate levels, achieving the lowest concentrations across all tests. In contrast, tap water exhibited the highest nitrate concentration, significantly exceeding that of the mycelium-treated samples. While both Phanerochaete chrysosporium and Trametes versicolor had positive effects on nitrate reduction, Pleurotus eryngii was the most effective and stable in improving water quality. This was most likely due to the different filtering capacities of the mycelium types, with each mycelium interacting differently with the nitrates in the water. Nitrates are commonly found in water due to runoff from agricultural areas or from natural processes, and they can be reduced through processes such as absorption, denitrification, or microbial activity.
ppm N
The observed variation in nitrate-nitrogen (Nitrate-N) concentrations across the water samples can be the cause of the distinct capabilities of each mycelium type in reducing nitrate levels. Nitrate-N, a common pollutant found in water sources, is often the result of agricultural runoff, wastewater, or natural nitrogen cycles. Its removal or reduction in water is influenced by processes such as microbial denitrification or direct absorption by filtering agents like mycelium. Pleurotus eryngii consistently demonstrated the most effective and stable reduction of Nitrate-N levels, likely due to its high capacity for assimilating nitrogen compounds into its biomass or through biological processes that promote denitrification. This efficiency may stem from its fungal structure, which facilitates nutrient uptake and metabolic activities that directly interact with nitrate ions. Phanerochaete chrysosporium and Trametes versicolor also contributed to nitrate reduction, with Trametes versicolor exhibiting the most stable and consistent performance. The reduction in nitrate levels by these mycelia can be attributed to their similar mechanisms of nitrogen absorption or microbial activity, although Phanerochaete chrysosporium showed some variability in its effectiveness, suggesting that its ability to process nitrates may be influenced by environmental factors or its specific growth patterns. Tap water, on the other hand, maintained high nitrate-nitrogen concentrations, reflecting its exposure to external sources of nitrate pollution, which were not effectively removed by the mycelium filters. These results underscore the potential of using mycelium, especially Pleurotus eryngii and Trametes versicolor, as efficient biological filters for reducing nitrate levels and improving water quality, particularly in environments exposed to agricultural or industrial runoff.
Limitations
General Limitations
- Mycelium filters slower than synthetic methods, making it less ideal for large-scale or high-demand applications
- Requires controlled temperatures to thrive; extreme heat or cold can hinder performance
- Difficult and expensive to scale for industrial use, requires optimal conditions to maintain effectiveness
- Mycelium is delicate and can break down under physical stress, making it unsuitable for long-term, high-demand filtration
- Biofilm can build up, clogging the system and reducing filtration efficiency, requiring regular maintenance
- Vulnerable to harsh chemicals, limiting use in environments with toxic substances
- Best for organic matter and heavy metals; struggles with non-organic pollutants or dissolved chemicals
- Mycelium degrades as it filters contaminants, reducing effectiveness and requiring replacement
- Inefficient at removing nitrates or inorganic contaminants, requiring complementary methods for these pollutants
- Competes with other microorganisms for nutrients, limiting its growth and filtration capacity
- Requires frequent upkeep to prevent clogging and maintain efficiency, adding operational costs
- Growing mycelium at scale is resource-intensive and costly, limiting economic feasibility for large-scale use
Phanerochaete Chrysosporium Limitations
- Bacteria: Effective at bacterial reduction but not specialized for breaking down organic pollutants
- Carbonate: Effective in reducing carbonate levels, but performance may vary in different environments
- pH: Minor fluctuations, lacks strong buffering effect compared to Pleurotus eryngii
- Lead: No measurable impact on lead removal, may require modification to filtration process
- Hardness: Moderately effective, but less efficient than Pleurotus eryngii in reducing water hardness
- EDTA After Adding: Some reduction in hardness but not as significant as Pleurotus eryngii
- EDTA Before Adding: Initial mineral content variations show it doesn’t drastically alter water hardness
- Phenolphthalein Alkalinity: Did not significantly alter alkalinity, lacks ability to change high alkaline water
- Bicarbonate Alkalinity: Slight reduction but less impactful than Pleurotus eryngii
- Total Alkalinity: Only moderate reduction, not as strong in lowering buffering capacity as Pleurotus eryngii
- Nitrate: Minimal nitrate absorption, not effective for nitrate removal compared to some plant-based filtration methods
Pleurotus Eryngii Limitations
- Bacteria: Less effective at bacterial removal compared to Trametes versicolor
- Carbonate: No significant effect on carbonate levels, showing minimal impact on mineral content
- pH: Only minor fluctuations, does not strongly buffer pH changes
- Lead: No significant effect on lead removal, may require alternative filtration methods
- Hardness: Most effective in reducing hardness but does not eliminate all calcium and magnesium ions
- EDTA After Adding: Most efficient at reducing hardness but still leaves residual minerals
- EDTA Before Adding: Initial variations show inconsistencies in its impact on water hardness
- Phenolphthalein Alkalinity: No significant effect on strong alkaline conditions
- Bicarbonate Alkalinity: Lowered alkalinity but does not eliminate buffering capacity entirely
- Total Alkalinity: Strongest reduction in alkalinity but does not completely neutralize buffering compounds
- Nitrate: Some nitrate reduction but not a primary function, effectiveness varies depending on environmental conditions
Trametes Versicolor Limitations
- Bacteria: Moderate reduction but not specialized for bacterial contamination removal
- Carbonate: Moderately reduces carbonate levels, though less efficient than Phanerochaete chrysosporium
- pH: Increases and stabilizes pH at a more alkaline level, which may not be ideal for all water sources
- Lead: No measurable impact on lead reduction, not suited for heavy metal filtration
- Hardness: Least effective among the three in reducing water hardness
- EDTA After Adding: Reduction in hardness but significantly less effective than Pleurotus eryngii
- EDTA Before Adding: Initial mineral content shows minimal effect on hardness reduction
- Phenolphthalein Alkalinity: No significant impact on altering strong alkaline conditions
- Bicarbonate Alkalinity: Maintains higher alkalinity, does not reduce buffering effect significantly
- Total Alkalinity: High total alkalinity, lacks ability to lower water’s buffering capacity effectively
- Nitrate: Poor nitrate removal capability, not ideal for nitrate filtration
Conclusion
Our hypothesis proposed that Pleurotus eryngii would be the most effective at filtering contaminants from water due to its enzymatic properties and strong adsorption capabilities, particularly for heavy metals like lead and other pollutants. The results of our experiment largely supported this prediction, as Pleurotus eryngii consistently demonstrated superior performance in removing lead and nitrate, often reducing them to undetectable levels. Additionally, it showed strong potential in lowering carbonate and total alkalinity levels, which further contributed to overall water quality improvements. However, while it performed exceptionally well in these areas, its ability to regulate pH and hardness showed some inconsistencies across trials. This suggests that while Pleurotus eryngii is highly effective in contaminant removal, its filtration performance may still be influenced by external environmental factors, growth conditions, or the specific composition of the contaminated water. Despite these minor fluctuations, our findings align with existing research, reinforcing the idea that Pleurotus eryngii mycelium has significant bioremediation potential and may be one of the most reliable mycelium species for water filtration applications. Moreover, our results highlight how the structural composition of mycelium plays a crucial role in determining its effectiveness. The dense, fibrous network of Pleurotus eryngii likely contributed to its superior adsorption capabilities, allowing it to trap and retain contaminants more efficiently than the other species. This suggests that further exploration of mycelial structure, particularly in relation to its porosity and surface area, could enhance our understanding of how different fungi perform under various filtration conditions.
Pleurotus eryngii emerged as the strongest candidate for effective filtration, particularly in its ability to remove lead, nitrate, and other impurities from contaminated water. Observations of its structure revealed dense, well-developed mycelial growth, which likely contributed to its filtration efficiency. Its adsorption capabilities, combined with enzymatic degradation of organic pollutants, allowed for a thorough purification process that outperformed the other species in multiple parameters. However, despite its strengths, Pleurotus eryngii was not without its limitations. Some trials showed fluctuations in pH stability and hardness levels, suggesting that while it is highly efficient in removing certain contaminants, its impact on overall water chemistry can be unpredictable. External factors such as temperature, nutrient availability, and potential microbial interactions could have influenced these inconsistencies. Nevertheless, Pleurotus eryngii’s strong overall performance makes it a promising candidate for future bioremediation research, with potential improvements focusing on enhancing stability and optimizing environmental conditions to ensure more consistent results. Additionally, its ability to form robust mycelial mats may have contributed to its ability to physically trap and sequester contaminants. This factor could be significant in future applications where scalability is a concern, as denser mycelium networks may be more effective in large-scale water treatment systems. Further studies investigating the relationship between mycelial density and filtration efficiency would provide valuable insights into optimizing this species for practical use in diverse environmental conditions.
Phanerochaete chrysosporium displayed significant filtration potential, particularly in its ability to reduce lead and nitrate concentrations while demonstrating relatively stable pH regulation across trials. Unlike Pleurotus eryngii, which showed fluctuations in pH and hardness, Phanerochaete chrysosporium appeared to maintain more consistent pH levels, suggesting that it may have a stronger buffering capacity. However, despite its success in contaminant removal, its performance in reducing hardness and total alkalinity varied across tests, with some trials showing drastic reductions and others yielding less significant changes. These inconsistencies indicate that while Phanerochaete chrysosporium is a strong candidate for water filtration, its overall effectiveness may depend on external conditions, including variations in water composition, environmental factors, or differences in mycelial growth patterns. Its enzymatic activity, known for breaking down complex organic materials, likely played a role in its filtration success, but further studies would be needed to determine how to optimize its performance for large-scale applications. Additionally, its moderate growth rate and structural differences compared to Pleurotus eryngii may have played a role in its filtration behavior. Unlike the dense mats formed by Pleurotus eryngii, Phanerochaete chrysosporium mycelium exhibited a slightly looser structure, which may have impacted its ability to trap certain contaminants effectively. Understanding how these structural differences influence filtration efficiency could provide deeper insights into how mycelium can be engineered for optimal bioremediation applications.
Trametes versicolor, though still effective in certain aspects, demonstrated the least consistent results among the three species tested. Its limited growth, as observed during the experiment, likely contributed to its reduced filtration capacity compared to Pleurotus eryngii and Phanerochaete chrysosporium. While it did show some ability to remove contaminants, its performance was highly variable, particularly in nitrate filtration, where fluctuations across trials suggested instability in its filtration mechanism. Hardness levels also displayed unpredictable reductions, with some tests showing a complete drop to 0 ppm, which could indicate an excessive depletion of minerals necessary for balanced water chemistry. Additionally, Trametes versicolor’s inconsistent performance may have been influenced by its slower growth rate or competition with other microorganisms present in the system. While this species has been studied for its potential in breaking down pollutants, our results suggest that it may require more specific growth conditions or additional structural support to achieve reliable filtration effects. Its weaker performance may also be linked to its natural ecological role, as Trametes versicolor primarily functions as a decomposer of lignin-rich materials in forest ecosystems. Unlike Pleurotus eryngii and Phanerochaete chrysosporium, which are known for their stronger enzymatic activity in degrading a wide range of contaminants, Trametes versicolor’s filtration capacity may be limited by its natural metabolic processes. Future studies could explore whether modifications to its growth conditions, such as nutrient supplementation or structural reinforcement, could improve its consistency and overall effectiveness in water filtration applications.
In conclusion, our findings support the effectiveness of mycelium-based filtration, with Pleurotus eryngii demonstrating the strongest overall performance in removing lead and nitrate while also contributing to improvements in carbonate and total alkalinity levels. Phanerochaete chrysosporium mycelium showed strong potential, particularly in maintaining pH stability, but its performance in hardness and total alkalinity was inconsistent. Trametes versicolor mycelium, though still capable of some filtration, was the least reliable due to its limited growth and fluctuating contaminant removal results. Despite some inconsistencies, all three species contributed to water purification, reinforcing the viability of mycoremediation as a natural filtration method. These results highlight the need for further research to optimize mycelium filtration systems, improve growth conditions, and enhance stability for practical, large-scale applications. Additionally, understanding the biochemical and structural factors that influence filtration efficiency will be essential in developing more reliable and effective bioremediation strategies. The variations observed in filtration performance emphasize the importance of tailoring mycelium species selection based on specific environmental conditions and target contaminants. Future studies could focus on optimizing growth conditions, exploring genetic variations within species, and testing additional fungal strains to further expand the potential of mycelium-based water filtration technologies.
Application
This project is important and applicable to the real world because it explores the issue of water pollution, which affects billions around the world each year. It provides insight on an alternative to traditional water filtration systems, which can assist in making clean more accessible to those in need, especially to those in third-world countries. Unlike traditional chemical water filtration systems, fungi are easy to grow and cost-effective. If proven to be an effective water filtration method, it could be a far more accessible and practical method to implement in areas with limited resources. Furthermore, mycelium’s potential to reduce dependency on expensive and chemical-based filtration processes reflects the demand for eco-friendly solutions in today’s society, making our project not only current but also valuable in advancing sustainable water management practices.
In water filtration, mycelium is used to break down and remove contaminants, especially organic matter, heavy metals, and biodegradable pollutants. It acts as a natural filter by absorbing or degrading harmful substances, improving water quality. Some mycelium species, like oyster mushrooms, have been found to filter toxins such as petroleum, dyes, and certain chemicals. Mycelium-based filtration systems are eco-friendly alternatives to traditional synthetic filters, as they are biodegradable and sustainable. However, their filtration rate can be slow, and they require regular maintenance to prevent clogging and degradation.
Additionally, our research addresses the growing interest in mycelium, a material recognized in recent years for its remarkable properties, aligning our project with current environmental and sustainability trends. Beyond water filtration, mycelium’s versatility spans across various industries. It’s biodegradable, quickly growing, and surprisingly strong, making it ideal for sustainable packaging, building materials, and textiles. A 2020 study from the University of Michigan even explored the use of mycelium as a sustainable material to replace plastics in packaging and construction. Researchers found that mycelium could offer a biodegradable, stronger, and eco-friendly alternative to plastic, capable of breaking down harmful substances while providing structural integrity. Mycelium has also been used in furniture and interior design, with companies such as Ecovative Design and Mogu producing compostable acoustic panels, lampshades, and furniture components. In fashion, brands like Mylo™ by Bolt Threads are creating mycelium-based leather alternatives, used by Stella McCartney and Adidas as sustainable, cruelty-free substitutes for traditional leather. Its fibrous structure is also being explored in plant-based meat alternatives, while its antimicrobial properties show promise in wound dressings and biodegradable medical implants. Additionally, mycelium’s soundproofing and insulating abilities make it an eco-friendly option for sustainable construction.Mycelium is also able to improve soil health by breaking down harmful contaminants while promoting the availability of nutrients, meaning it has the potential to repair damaged ecosystems.
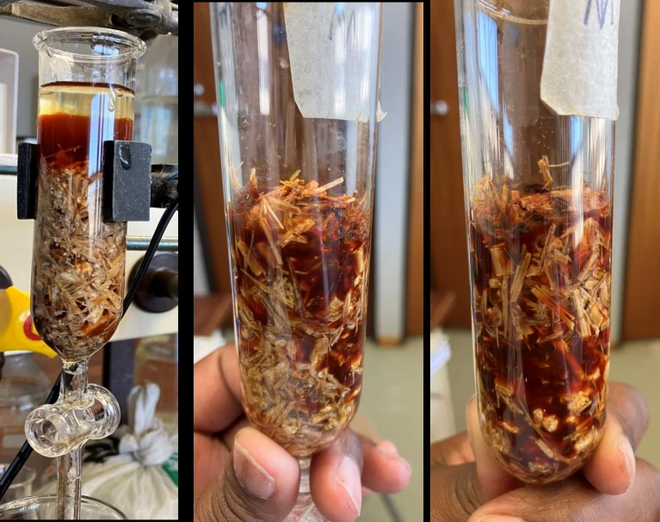
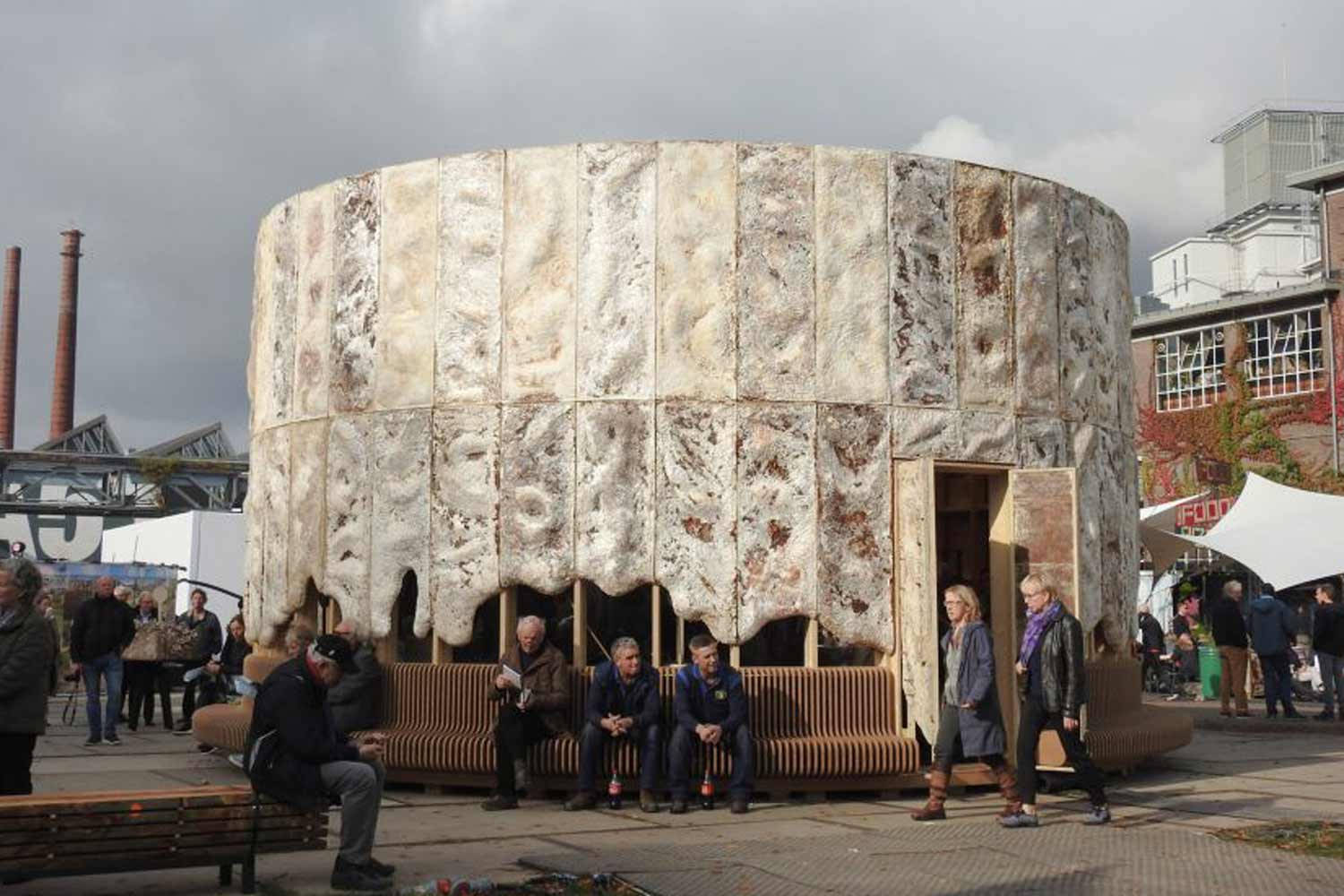
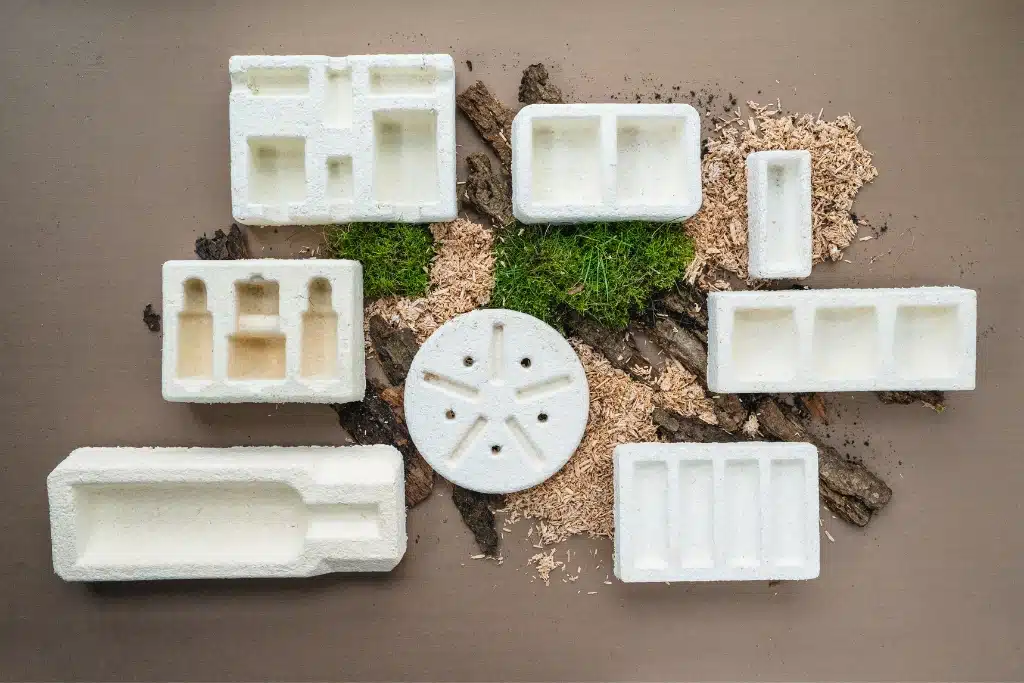
Sources Of Error
Citations
APA format:
- Stametes, P. (n.d.-b). Mycelium running: How mushrooms can help save the world. https://library.uniteddiversity.coop/Permaculture/Mycelium_Running.pdf
- Mnkandla, S. M., & Otomo, P. V. (2021a, July 28). Effectiveness of mycofiltration for removal of contaminants from water: A systematic review protocol - environmental
- evidence. BioMed Central. https://environmentalevidencejournal.biomedcentral.com/articles/10.1186/s13750-021-00232-0
- Rita, V. (2024a, October 15). Edible mushrooms help to purify contaminated water. Nature News. https://www.nature.com/articles/d44148-024-00294-5
- Iberdrola. (2021, April 22). Water pollution: How to protect our source of life. Iberdrola. https://www.iberdrola.com/sustainability/water-pollution
- Mehta, A., Dubey, R., & Kumar, S. (n.d.). (PDF) mycofiltration: A step towards sustainable environment. https://www.researchgate.net/publication/317618700_Mycofiltration_A_Step_Towards_Sustainable_Environment
- Agrios, G. N. (2014). Mycelium. Mycelium - an overview | ScienceDirect Topics. https://www.sciencedirect.com/topics/agricultural-and-biological-sciences/mycelium#chapters-articles
- Alberta Health Services. (2023). Contaminated water advisory remains in place for Elbow River (within the City of Calgary). https://www.albertahealthservices.ca/news/Page17517.aspx
- Natural Resources Defense Council. (n.d.). Water pollution: Everything you need to know. Natural Resources Defense Council. https://www.nrdc.org/stories/water-pollution-everything-you-need-know
- Dixon, T. (2011). The impacts of land use change on water quality in the Georgia Piedmont. (Master's thesis). Georgia State University. https://scholarworks.gsu.edu/cgi/viewcontent.cgi?article=1172&context=geosciences_theses
- Wright, M. R., & Lonsdale, W. M. (2000). The role of fire in the management of invasive plant species in the United States. USDA Forest Service, Rocky Mountain Research Station. https://www.fs.usda.gov/rm/pubs/rmrs_p072/rmrs_p072_023_028.pdf
- Incredible Mushrooms. (n.d.). Questions about using mushrooms for desalination and gray water filtration. Incredible Mushrooms.https://www.incrediblemushrooms.com/questions-about-using-mushrooms-for-desalination-and-gray-water-filtration.html#:~:text=To%20set%20up%20a%20mycofiltration,collected%20and%20used%20for%20irrigation
- WikiHow. (n.d.). How to create a mycofiltration system. WikiHow. https://www.wikihow.life/Create-a-Mycofiltration-System
- Author(s). (2024). Title of the article. ScienceDirect. https://doi.org/10.1016/j.sciadv.2024.01.001
- Author(s). (2024). Title of the article. Undergraduate Research Symposium. Kennesaw State University. https://digitalcommons.kennesaw.edu/undergradsymposiumksu/spring2024/spring2024/63/
- Mallak, A. M., Lakzian, A., Khodaverdi, E., Haghnia, G. H., & Mahmoudi, S. (2020). Effect of Pleurotus ostreatus and Trametes versicolor on triclosan biodegradation and activity of laccase and manganese peroxidase enzymes. Science of The Total Environment, 740, 139930. https://doi.org/10.1016/j.scitotenv.2020.139930
- Kennesaw State University. (2024). Effect of Mycofiltration on Water Pollution: A Study on Fungal Filtration Properties. Kennesaw State University Undergraduate Research Symposium. https://digitalcommons.kennesaw.edu/undergradsymposiumksu/spring2024/spring2024/63/
- Otomo, P. (2024, October 18). Fungal mycelia research offers potential for SA’s water crisis. Engineering News. https://www.engineeringnews.co.za/article/fungal-mycelia-research-offers-potential-for-sas-water-crisis-2024-10-18
- HomeWater. (2023, October 18). The hard facts about Calgary water quality. HomeWater. https://www.homewater.com/blog/the-hard-facts-about-calgary-water-quality
- Government of Alberta. (2004). Impacts of water quality in the Upper Elbow River [PDF]. Alberta Environment. https://open.alberta.ca/dataset/321b2ca3-4aeb-4734-b151-20a18dd18955/resource/21c99e4c-20d9-4d5e-8f11-820cf98faae8/download/impactswaterquality-upperelbowriver-2004.pdf
- Tavakkol, Z., & Mahdavi, M. (2006). Mycoremediation of water contaminated with heavy metals using fungal biomass. Applied Environmental Microbiology, 72(11), 7056–7063. https://pubmed.ncbi.nlm.nih.gov/16838717/
- Akin, R. A. (2019). The role of mycelium in bioremediation of environmental contaminants (Master's thesis, Georgia State University). Georgia State University ScholarWorks. https://scholarworks.gsu.edu/cgi/viewcontent.cgi?article=1172&context=geosciences_theses
- Kirk, P. M., & Lücking, R. (2015). Mycelium as a tool for bioremediation of environmental contaminants: An overview. Science of The Total Environment, 537, 9–24. https://doi.org/10.1016/j.scitotenv.2015.01.062
Photo sources:
-
Mayne Island Conservancy. (n.d.). Mycelium: The highway under the soil. Retrieved from https://mayneconservancy.ca/mycelium-the-highway-under-the-soil/
-
Royal Botanic Gardens, Kew. (n.d.). Fungi: The hidden dimension. Retrieved from https://www.kew.org/read-and-watch/fungi-hidden-dimension
-
ResearchGate. (2023). Interactions between bacteria and mushroom mycelium: Bacteriolytic enzyme production. Retrieved from https://www.researchgate.net/figure/nteractions-between-bacteria-and-mushroom-mycelium-a-Bacteriolytic-enzyme-production_fig2_367020926
-
ResearchGate. (2022). Schematic diagram illustrates water contamination due to natural sources, droughts, and pollution. Retrieved from https://www.researchgate.net/figure/Schematic-diagram-illustrates-water-contamination-due-to-natural-sources-droughts-and_fig2_362405344
-
Ghosh, S., & Ghosh, S. (2021). Mycelium-based composites for sustainable packaging solutions. In Mycelium composites: Emerging sustainable materials (pp. 101-119). Springer. https://link.springer.com/chapter/10.1007/978-981-16-0045-6_6
-
Parametric Architecture. (2023). From fungi to foundations: Mycelium in construction. Retrieved from https://parametric-architecture.com/from-fungi-to-foundations-mycelium-in-construction/
-
Sustainable Food Trust. (2022). The fungi thing about soil. Retrieved from https://sustainablefoodtrust.org/news-views/fungi-thing-about-soil/
Acknowledgement
We would like to acknowledge and thank everyone who provided their help, support, and feedback throughout this process. First and foremost, we extend our gratitude to our parents, whose encouragement and assistance have been extremely valuable. We are also grateful to our teacher, Mrs. Ball, and Mrs. Davis, for their continuous guidance and support throughout our entire project. Additionally, we would like to express our sincere appreciation to Professor Lisa Gieg and Fran Cusack from the University of Calgary for generously providing the mycelium culture and offering their expertise, which played a crucial role in the success of our research. We would also like to acknowledge all of the experts we contacted, such as Dr. Lloyd R. Wilson, and also the ones who weren't able to get back to us. Lastly, we would like to extend a special thanks to Daniel Larson, who along with Fran Cusack, volunteered their valuable time and tested our samples at the University of Calgary's lab facilities, as well as Louis Riel school and CYSF for this exciting opportunity.